
Halophiles
Biology
Halohpilic microorganisms are fascinating specimens of biology because of their ability to thrive where other organisms may not. The predisposition they have toward higher salt concentrations is attributed to various adaptations that have been examined to a generous extent due to their uniqueness and potential for application in the developing world of biotechnology.

Nutrition
Microorganisms of the order Halobacteriales utilize carbon sources through the tricarboxylic acid cycle (Fig. 1), sometimes in cooperation with the glyoxylate cycle, and an electron transport chain comprising various cytochromes. The nutritional demands differ greatly amongst genera and species alike, however the consensus seems that most halophilic Archaea prefer amino acids as a carbon and energy source. e.g. and sp. NRC-1. They can be recycled back into TCA cycle and then be utilized genes expressing proteins for a reverse glycolysis pathway required for gluconeogenesis (Fig. 2) [8].
Figure 1
This depicts the general biology of H salinarum NRC-1, showing its ability to uptake nutrients, produce energy and transports cations/anions.
Image obtained from Genome sequence of Halobacterium species NRC-1 [8]
Figure 2
Image depicting gluconeogenesis and which amino acids can enter this pathway and from which point.
Hypersaline solutions have a lower affinity for non-polar oxygen and therefore minimal oxygen concentrations are common. Many halophillic archaea have ability to respire anaerobically, requiring the archaea to use a variety of different final electron acceptors: Haloferax denitrificans uses nitrogen, however since nitrates exist in low concentrations and are not recycled by nitrification, the pathway is of limited application. H. salinarum can use dimethylsulfoxide, trimethylamine N-oxide and fumarate [9].
H. salinarum expressing bacteriorhodopsin genes may also survive in almost anaerobic conditions, however oxygen is required for bacteriorhodopsin biosynthesis and therefore a minimum level must be present [3].
Light Utilizing Proteins
Under the microscope a visible trait of Halobacteriales is their bright red-orange colour attributed to the incidence of carotenoid pigments in the membrane of these halophiles [3]. Halobacteriales are known to contain at least four retinal-containing proteins; bacteriorhodopsin, halorhodopsin, and two sensory rhodopsins linked with phototaxis.
Bacteriorhodpsin, is a retinal based pigment that carries out light mediated transport of protons, for energy conservation, its high content in the sea may indicate varying oxygen content [5]. It consists of several components; opsins a transmebrane complex consisting of 7 helices, chromophore retinal responsible for colouration [5].
The two are bonded to one another resulting in protonated Schiff base linkage (functional group: R-NH+=C) [5]. It appears purple when protonated. Thus purple membrane name.
Photon absorption by the retinal causes the movement of the proton along the chromophore-opsin complex eventually resulting in the proton leaving the complex at the extracellular side. The process creates proton electrochemical gradients that can be used to generate ATP [13].
This diagram demonstrates the process of chemiosmosis, illustrating the pigment pumping out protons and allowing them back through ATP-synthase complexes generating ATP from the energy.
Bacteriorhodopsin can also be linked with sustained exit of Na+ from the cell via the Na+-H+ antiport system, ensuring the correct levels of K+ actively transported into the cell using ATP as an energy source and indirectly assisting uptake of amino acid through a cotransport system with Na+ [7].
Halorhodopsin, was shown by Schobert and Lanyi to pump chloride ions into the cell from the external media following excitation by light. This is essential for maintaining cell growth and osmotic balance with the outside environment. H. salinarum also possesses sensory rhodopsins for phototaxis. Sensory rhodopsin I promotes movement via flagella toward green light in H. salinarum whilst sensory rhodopsin II receives blue light and advocates movement away from this stimulus [3].
Bacterioruberin a C50-carotenoid is thought to help protect the cell against UV damage [12].
Adaptions
Salt Adaptation
Extreme halophiles maintain osmotic balance by importing K+ to match extracellular osmotic concentration. Other halophiles (non-extreme) maintain balance by producing “compatible solutes” such as amino acids and variant forms of sugars. They can also be taken up from the environment. These conditions would cause denaturation in non halophillic proteins. Clearly extreme halophiles require proteins that are adapted to these salt conditions [2]. The 3D shape of a protein is dependent on the interactions between surface of the protein with itself, other proteins, water and solutes (salt); maintaining solubility, stability, shape and thus activity [11].
Halophillic proteins and enzymes show an acidic amino acid residue preference, namely aspartate and glutamate, the later has particularly good water binding properties [6]. Aswell as a lack of lysine and other basic amino acids.
No conclusive role has been determined for the acidic residues on halophillic proteins, however two have been suggested. The negative charge either directly competes with salt ions for water, or binds to hydrated cations allowing a hydrated layer to form around the protein [11] important for solubility, and maintenance of structure related activity aswell as stabilising against aggregation and unfolding [10]. Gerald Bohm and Rainer Jaenicke, suggested these traits caused electrostatic repulsion between different regions of the protein which are unfavourable for stability, and that high concentrations of salt could reduce these effects due to binding [1].
Image depicts the surface of the glucose dehydrogenase protein from the extreme halophile, Haloferax mediterranei, the red areas represent surface potential difference less than -10.
Halophillic proteins have “Less hydrophobic contact” in their core. This balances out the strength of such hydrophobic bonds in high salinity. Meaning aggregation and thus a loss of function is less likely.
UV Light Adaption
Excessive UV light causes genetic mutation by promoting the formation of pryrimidine dimers (TT). Halophiles require large amounts of DNA repairing proteins aswell as carotenoids (Bacterioruberin) which provide photoprotection as well as being a very essential antioxidant. DNA recombination is another feature which enables cells combat DNA damaged. Having a low number of thymine molecules is crucial in halophilic cells, due to them being UV “targets”.
Shape/Morphology
Halobacteriaceae exhibit an assortment of shapes:
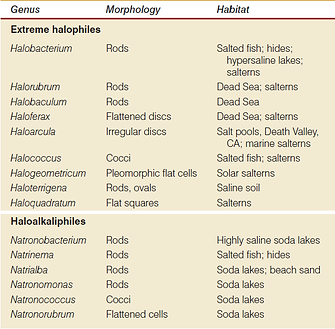
The variety of shape can be credited to the lack of significant turgor pressure in such microorganisms [9].
Excluding the non-coccoid cells, haloarchaeal morphology changes in a salt concentration-dependent manner [4]. This is related to the cell wall ion-requirement for stability. Aswell as the glycoprotein composed S-layer/cell wall layer of Halobacteriaceae which maintains shape.
Halobacteriales also have flagella present. H. salinarum makes bundles of flagellin filaments which are constructed from sulphated glycoproteins.
The flagella of halophilic Archaea have right-handed helices in contrast to Bacterial flagella that have left-handed helices, therefore clockwise rotation permits forward movement and vice versa. Movement stimulated by chemo and photo sensors [9].
Gas vesicles are found in members of Halobacteriaceae, they benefit an aerobic host by orchestrating floatation to the surface of the organism’s environment, allowing it to take advantage of a vertical oxygen gradient when oxygen is in low supply. The genes coding this trait are lost with high frequency, perhaps as a result of the genes usefulness being dependent on environmental conditions [9], [3].
References
-
Bohm, G. and Jaenicke, R. (1994). A structure-based model for the halphilic adaptiation of dihydrofolate reductase from Halobacterium volcanii. Protein Eng Des Sel, 7(2), pp.213-220.
-
Britton, K., Baker, P., Fisher, M., Ruzheinikov, S., Gilmour, D., Bonete, M., Ferrer, J., Pire, C., Esclapez, J. and Rice, D. (2006). Analysis of protein solvent interactions in glucose dehydrogenase from the extreme halophile Haloferax mediterranei. Proceedings of the National Academy of Sciences, 103(13), pp.4846-4851.
-
Dworkin, M. and Falkow, S. (2006). . New York: Springer.
-
Fendrihan, S., Legat, A., Pfaffenhuemer, M., Gruber, C., Weidler, G., Gerbl, F. and Stan-Lotter, H. (2006). Extremely halophilic archaea and the issue of long-term microbial survival. Reviews in Environmental Science and Bio/Technology, 5(2-3), pp.203-218.
-
Horikoshi, K. and Grant, W. (1998). Extremophiles. New York: Wiley-Liss.
-
Karan, R., Capes, M. and DasSarma, S. (2012). Function and biotechnology of extremophilic enzymes in low water activity. Aquat Biosyst, 8(1), p.4.
-
Lanyi, J. (1978). Light Energy Conversion in Halobacterium halobium. Microbiological Reviews, 42(4), pp.682-706.
-
Ng, W., Kennedy, S., Mahairas, G., Berquist, B., Pan, M., Shukla, H., Lasky, S., Baliga, N., Thorsson, V., Sbrogna, J., Swartzell, S., Weir, D., Hall, J., Dahl, T., Welti, R., Goo, Y., Leithauser, B., Keller, K., Cruz, R., Danson, M., Hough, D., Maddocks, D., Jablonski, P., Krebs, M., Angevine, C., Dale, H., Isenbarger, T., Peck, R., Pohlschroder, M., Spudich, J., Jung, K., Alam, M., Freitas, T., Hou, S., Daniels, C., Dennis, P., Omer, A., Ebhardt, H., Lowe, T., Liang, P., Riley, M., Hood, L. and DasSarma, S. (2000). Genome sequence of Halobacterium species NRC-1. Proceedings of the National Academy of Sciences, 97(22), pp.12176-12181.
-
Oren, A. (2002). Halophilic microorganisms and their environments. Dordrecht: Kluwer Academic.
-
Qvist, J., Ortega, G., Tadeo, X., Millet, O. and Halle, B. (2012). Hydration Dynamics of a Halophilic Protein in Folded and Unfolded States. J. Phys. Chem. B, 116(10), pp.3436-3444.
-
Reed, C., Lewis, H., Trejo, E., Winston, V. and Evilia, C. (2013). Protein Adaptations in Archaeal Extremophiles. Archaea, 2013, pp.1-14.
-
Shahmohammadi, H., Asgarani, E., Terato, H., Saito, T., Ohyama, Y., Gekko, K., Yamamoto, O. and Ide, H. (1998). Protective Roles of Bacterioruberin and Intracellular KCl in the Resistance of Halobacterium salinarium against DNA-damaging Agents. JRR, 39(4), pp.251-262.